Research Article
A Thermodynamic Study of the ChargeTransfer Complexes of [60] Fullerene with Thiapodands
Zarour AA1, Albedwani M1, Ashram1* and Mizyed2*
1Department of Chemistry, Mutah University, Al-Karak, Jordan
2Department of Chemistry, Yarmouk University, Irbid, Jordan
Corresponding author: Shehadeh Mizyed, Department of Chemistry, Yarmouk University, Irbid, Jordan, Tel: +962 790862295; E-mail: mizyed@yu.edu.jo
Citation:Zarour AA, Albedwani M, Ashram, Mizyed. A DFT Computational Study of the Antioxidant Activities Exhibited by 3-aryl-4-hydroxycoumarin 02 Derivatives. J Chem Applied Biochem. 2017;4(1): 120.
Copyright © 2017 Zarour AA, et al. This is an open access article distributed under the Creative Commons Attribution License, which permits unrestricted use, distribution, and reproduction in any medium, provided the original work is properly cited.
Submission: 14/01/2017; Accepted: 23/03/2017; Published: 14/04/2017
Abstract
Formation of charge-transfer complexes between thiapodands1-6 and [60]fullerene, C60, were studied in toluene solution using UV-Vis spectrophotometry. The stability constants and the thermodynamic data of the resulting 1:1 complexes were determined. For thiapodands with ester groups ending, the order of stability constants of [60]fullerene-podands, 1-3 is in the order 1>2>3 while for thiapodands with amide groups ending, the order of stability constants of [60] fullerene-podands, 4-6 is 6>5>4.
Keywords: [60] fullerene; Thiapodands; UV-Vis spectrophotometry; Complexes
Introduction
Fullerene, C60 represents the third crystallographic form of carbon after diamond and graphite. The high electron accepting properties of C60 and the high electron donating properties of some electron donors led to study the interaction between C60 and different types of electron donors. As an example, the complexation between C60 and calyx [n] arenes and calixarene derivatives was studied extensively [1-15]. In these studies, UV and NMR spectroscopy were used to establish the stoichiometry of the complexes formed between C60 and calixarene derivatives, the stability constants and the thermodynamic parameters were also determined. Also C60 is known to form complexes with different types of crown ethers [16-18]. Some of crown ethers formed 1:1 C60: crown complexes and others formed 1:2 C60: crown complexes [16-18]. The stability of the C60: crown complexes whether it is 1:1 or 1:2 complexes appears to be related to many factors including among others, the macrocyclic cavity size and the type of donor atoms at the periphery of crown moiety. The stability would be a maximum when the cavity size matches the guest size [19,20]. In addition, substitution of one or more oxygen atoms by sulfur or nitrogen atoms enhances the complexation ability of the hosts [19,20].
Recently, Ashram reported the synthesis of thiapodands 1-6 (Scheme 1) [20]. These podands have different cavity sizes and different donor atoms including O, N and S atoms. The ability of these podands to form complexes with some transition metals in addition to their stability constants and thermodynamic properties were reported [20].
As mentioned previously, most studies on the complexation of [C60] fullerene were done with cyclic hosts. To the best of our knowledge, there are no reports on the complexation of [C60] fullerene with podands.
In the present study, the determination of the stiochiometriy, stability constants and the thermodynamic parameters (ΔH°, ΔS°, and ΔG°) of thiapodands 1-6 complexes [60]fullerene, using UV-Vis spectrophotometry is reported.
Results and Discussions
When a violet solution of C60 in toluene is mixed with any colorless solution of podands 1-6, a pale yellow solution is developed. This change in color indicates the formation of a charge transfer complexes between these podands and C60. The absorption spectra of a series of solutions of podand1 and C60, as an example, are shown in Figure 1.
Figure 1 reveals that the resulting complex has a new absorption band at λ =435 nm. None of the host or the guest shows any measurable absorption at 413-455 nm region. Thus the existence of this new band must be associated with the formation of donoracceptor molecular complex between podand1and C60 in solution. Mukherjee et al. reported the formation of similar charge transfer absorption bands of the complexes of calyx [6] and calyx [8] arene derivatives with [60]fullerene [21]. Stoichiometries of all podands-C60 complexes were determined to be 1:1 by mole ratio method and confirmed by using Job’s method. Figure 2 shows the mole ratio plot for the complex of podand 1 with C60. The change in the slope near the 1:1 ratio is clearly shown, this indicates the formation of 1:1 complex. This stoichiometry is confirmed by Jobs plot which is shown in Figure 3 for the same system. The figure shows a maxima at 0.5 which confirms the formation of 1:1 complex. Complexes of C60 with other podands show similar behavior.
Supramolecular 1:1 complex formation between podand 1, as an example, with C60 in solution can be represented by equation 1.
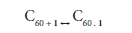
The corresponding formation constant Kc (stability constant) for1 is given by equation 2
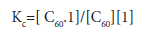
Since both podand1 and [60 ]fullerene concentrations are very low, the stability constant Kc, can be calculated by using the following modified form of the Benesi-Hildebrand equation 3 [22]:

where [C60]o is the initial concentration of C60, Kc the formation constant, ΔA the absorbance change at λ = 435 nm of C60 solution in toluene upon the successive addition of thiapodand1, Δε represents the difference in the molar extinction coefficient between the C60.1 complex and that of uncomplexed C60. When [C60]o /ΔA is plotted against 1/ [1]o gives a straight line with a slope of 1/εKc and intercept of 1/ε is obtained as shown in Figure 4. Kc value in each case is calculated by dividing the intercept by the slope (determined bylinear regression analysis according to equation 3).
In order to achieve a better understanding of the thermodynamicsof thiapodands-[60]fullerene complexation reactions, it would be useful to consider the enthalpic and entropic contributions to these complexation processes. These thermodynamic parameters (ΔG°, ΔH° and ΔS°, Table 1) were evaluated from the temperature-dependence of the stability constants (Kc) and applying the van’t Hoff equation.

A plot of lnKc against 1/T gives a straight line with -ΔH°/ R as a slope, and ΔS°/R as an intercept as shown in Figure 5 for C60 podand 1 complex. All other complexes of thiapodands with C60 give similar linear plots.
Table 1 lists the stability constant values determined at 25°C and thermodynamic parameters ΔHo, ΔSo and ΔGo values for the chargetransfer complexes formed between C60 and thiapodands 1-6.
Generally, the stability constant value is an indication about thestability of the complex between donor and acceptor, i.e the strength of acceptor-donor interaction. The larger the value, the higher is the stability of the complex. Many factors influence the stability constants, among them; the size of the guest, number and type of the donor atoms and a macrocyclic effect which involves the dimensional compatibility between the size of the macrocycle and the size of the guest. Results in Table 1, show the order of stability constants of C60 and ester group pearing thiapodands 1-3 complexes is in the order of 1>2>3. This behavior of the podands can be explained by considering the cavity sizes of the podands and the ligating heteroatoms, oxygen and sulfur atoms at the periphery of the podands. The higher stability of the complex of 1 over the complexes of 2 and 3 could be due to the cavity size ratio of podand to [60]fullerene that gives more proper spatial orientation for the interaction, despite the larger number of heteroatoms in each of 2 and 3 as compared to 1. The replacement of one of the sulfur atoms in podand 2 with oxygen atom gives podand 3 causes a decrease in the stability constant. This is because sulfur atom is better donating base toward neutral acceptors than oxygen atom [23-25]. For C60 and amide group pearing thiapodands 4-6 complexes; the order of stability constants is in the order of 6 >5 >4. The situation here is completely reversing the order of stability constants in C60- thiapodands 1-3 complexes. This dramatical change could be due to changing the nature of the ending groups; from ester ending groups to amide ending groups. Previous studies on the complexation of podands 1-6 with some transition metals showed clear evidence that the nature of the ending groups takes part in determining the value of stability constants [19,20].
Table 1 also shows that podands 1 and 2 complexes are enthalpy stabilized and entropy destabilized while podands 3, 4, 5 and 6 are enthalpy destabilized, but are highly entropy stabilized.
The less negative ΔH° value of 3:[60]fullerene complex as compared to 1 and 2:[60]fullerene complexes indicates weaker interaction between [60]fullerene and podand 3 compared to that between [60]fullerene and podands 1 or 2. This probably due to the donation power of the softer sulfur atoms over the harder oxygen atoms.
The extent of flexibility is expected to vary with the size of the podand due to the number and the type of atoms at the periphery of the podands. The interaction of a C60 molecule with a flexible podand results in the formation of a less flexible charge-transfer complex. This will lead to a negative ΔS° values for the complexation process. It is known that the most flexible starting host will form a complex with the most negative ΔS° value. This explains the most negative ΔS° value for the 2:[60]fullerene complex as compared to podands 1 and 3.
For comparison purposes, the stability constants of some macrocyclic compounds 8-14 (Scheme 2) with C60 were incorporated (Table 2). It is clear that the stability constants of podands 1-6 are larger than the stability constants of compounds 8-14 macrocylic-C60 complexes.
Experimental
Thiapodands 1-6 were prepared according to the published procedures [20]. Toluene (HPLC grade, GCC, assay 99.8%) and [60]fullerene (99.5%, Aldrich) were used without any further purification. Spectrophotometric measurements were carried out using a UVspectrophotometer (SHIMADZU), Model UV-2450, equipped with a thermostat cell holder. The temperature controller (SHIMADZU) was provided with a cooling facility and used to control the cell temperature in the range (0.00-80.0°C) with an accuracy of ± 0.1°C.
The concentration of [60]fullerene in each solution was kept constant at 1.30 × 10-4 M, and podands 1-6 concentrations were increased from 0.00 up to 8.00 ×10-3 M. Three runs were made for the calculation of certain K value. Mole ratio method was used to determine the stoichiometry for the complexes. Job’s method was applied to confirm the 1:1 stoichiometry of the complexes [15]. The stability constants were evaluated at various temperatures (15-35°C)using Bensesi-Hildebrand equation and van’t-Hoff equation was applied to determine the thermodynamic parameters [22].
Conclusion
The present study shows that the donors 1-6 formed 1:1 complexes with C60 in Toluene solution. In each case, the complex formation was confirmed by the change in the UV-Vis spectra. Several factors affected the complex formation such as, the cavity size, the type and the number of donor atoms and the type of the ending group. It was noticed that, the Kc values increased as the cavity size decrease when the ending group is ester group while the opposite is also true when the amide group is the ending group. The order of stability constants when the ester is the ending group is in the order of1>2>3, while the order of stability is 6>5>4when the amide is the ending group.
Acknowledgment
The author M. Ashram would like to thank the Mutah University(Jordan) for the support offered during the course of this research.
References
- Andersson T, Nilsson K, Sundahl M, Westman G, Wennerstrom O (1992) C60 embedded in γ-cyclodextrin: A water-soluble fullerene. J Chem Soc Chem Commun 8: 604-606.
- Williams RM, Verhoeven JW (1992) Supramolecular encapsulation of C60 in a water soluble calixarene; A core-shell charge transfer complex. Recl Trav Chim Pays Bas 111: 531-532.
- Ikeda A, Yoshimura M, Tani F, Naruta Y, Shinkai S (1998) Construction of a Homooxacalix[3]arene-based dimeric capsule cross-linked by a Pd(II)-pyridine interaction. Chem Lett 27: 587-588.
- Ikeda A, Udzu H, Yoshimura M, Shinkai S (2000) Inclusion of [60]fullerene in a self-assembled Homooxacalix[3]arene-based dimeric capsule constructed by a PdII-pyridine interaction. The Li+-binding to the lower rims can improve the inclusion ability. Tetrahedron 56: 1825-1832.
- Steed JW, Junk PC, Atwood JL, Barnes MJ, Raston CL, et al. (1994) Ball and socket nanostructures: New supramolecular chemistry based on cyclotriveratrylene. J Am Chem Soc 116: 10346-10347.
- Komatsu N (2003) Preferential precipitation of C70over C60 with p-halohomooxacalix[3]arenes. Org Biomol Chem 1: 204-209.
- DudiÄ M, Lhoták P, Stibor I, PetÅ™ÃÄková H, Lang K (2004) (Thia)calix[4]arene-porphyrin conjugates: Novel receptors for fullerene complexation with C70over C60 selectivity. New J Chem 28: 85-90.
- Atwood JL, Steed JW (2004) Encyclopedia of supramolecular chemistry. Dekker M, New York 1-872.
- Atwood JL, Barbour LJ, Nichols PJ, Raston CL, Sandoval CA (1999) Symmetryâ€aligned supramolecular encapsulation of C60: [C60⊂(L)2], L=pâ€Benzylcalix[5]arene or p-Benzylhexahomooxacalix[3]arene. Chem Eur J 5: 990-996.
- Atwood JL, Koutsoantonis GA, Raston CL (1994) Purification of C60 and C70by selective complexation with calixarenes. Nat 368: 229-231.
- Atwood JL, Bott SG, Jones C, Raston CL (1992) Aluminium-fused bis-p-tert-butylcalix[4]arene: A double cone with two π-arene ⋯ H interactions for included methylene chloride. J Chem Soc Chem Commun 1349-1351.
- Suzuki T, Nakashima K, Shinkai S (1994) Very convenient and efficient purification method for fullerene (C60) with 5,11,17,23,29,35,41,47-Octa-tert-butylcalix[8]arene-49,50,51,52,53,54,55,56-octol. Chem Lett 23: 699-702.
- Haino T, Yanase M, Fukazawa Y (1997) New supramolecular complex of C60 based on Calix[5]arene-Its structure in the crystal and in solution. Angew Chem Int Ed 36: 259-260.
- Mizyed S, Ashram M, Miller DO, Georghiou PE (2001) Supramolecular complexation of [60]fullerene with hexahomotrioxacalix[3]naphthalenes: A new class of naphthalene-based calixarenes. J Chem Soc Perkin Trans 2: 1916-1919.
- Mizyed S, Georghiou PE, Ashram M (2000) Thermodynamic study of the complexes of calix[4]naphthalenes with [60]fullerene in different solvents. J Chem Soc Perkin Trans 2: 277-280.
- Bhattacharya S, Sharma A, Nayak SK, Chattopadhyay S, Mukherjee AK (2003) NMR study of complexation of crown ethers with [60]- and [70]fullerenes. J Phys Chem B 107: 4213-4217.
- Ashram M, Maslat A, Mizyed S (2009) Synthesis and biological activities of new azacrown ether Schiff-bases and spectrophotometric studies of their complexation with [60]fullerene. Toxicol Environ Chem 91: 1095-1104.
- Mizyed S, Aleteiwi I, Marji D (2014) A thermodynamic study of the charge transfer complexes of C60 and some macrocyclic ligands. J Chem Appl Biochem 1.
- Mizyed S, Ashram M (2011) Synthesis and metal ion complexation of acyclic Schiff base podands with lipophilic amide and ester end groups. J Incl Phenom Macrocycl Chem 70: 135-142.
- Ashram M, Mizyed S, Zereini W, Rawashdeh AM (2012) Synthesis, metal ion complexation and antibacterial activities of some thiapodands with lipophilic amide and ester end groups. J Sulfur Chem 33: 561-571.
- Bhattacharya S, Nayak SK, Chattopadhyay S, Banerjee M, Mukherjee AK (2001) Spectrophotometric and thermodynamic study of supramolecular complexes of [60]- and [70]fullerenes with a number of calix[n]arenes. J Chem Soc Perkin Trans 2 2: 2292-3397.
- Benesi HA, Hildebrand JH (1949) A spectrophotometric investigation of the interaction of iodine with aromatic hydrocarbons. J Am Chem Soc 71: 2703-2707.
- Mizyed SA, Ashram M, Saymeh R, Marji D (2005) A thermodynamic study of the charge transfer complexes of iodine with different tert-butylcalix[4]crowns. Z Naturforsch b60: 1133-1137.
- Shamsipur M, Mashhadizadeh MH (2000) Spectrophotometric study of complex formation between iodine and some thiacrown ethers in chloroform solution. J Incl Phenom Macrocycl Chem 38: 277-286.
- Mizyed SA, Al-Jarrah E, Marji D, Ashram M (2007) A spectrophotometric study of the charge transfer complexes of [60]fullerene with different tert-Butylcalix[4]crowns. Spectrochim Acta A Mol Biomol Spectrosc 68: 1274-1277.